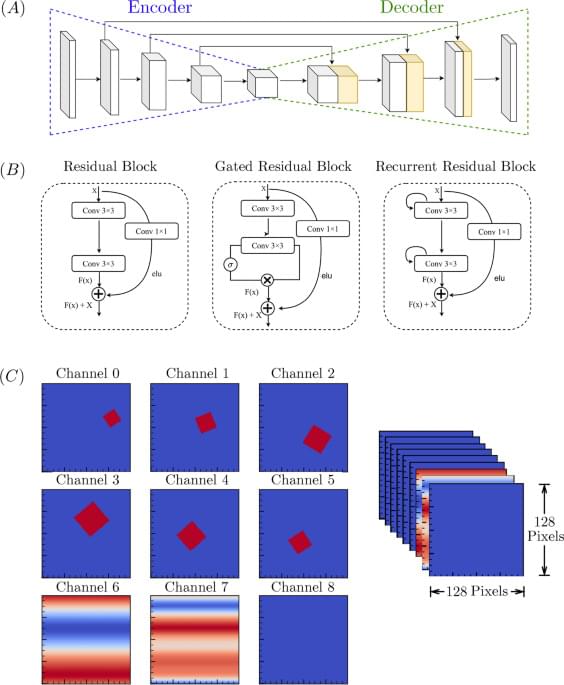
While machine learning methods can be used for accurate flow prediction in complex environments, such as for urban structures30 or turbulent fields31, generalizing these approaches to domains of arbitrary size and complexity remains a challenging problem. One reason is that flows near and around obstacles depend on factors associated with the fluid (i.e., Reynolds number) or domain (i.e., boundary conditions), and fixing either of these conditions puts bounds on the validity of the estimated fields. Thus, if we seek broad applicability, then we should seek the fewest set of model restrictions that together provide the most accurate flow predictions. To this end, our approach has been to deconstruct certain types of domains into individual obstacles that each maintain some level of geometrical similarity, so that a single neural network model can be used to predict flows near all structural boundaries of the domain. Flows between these structural surfaces, at a scale on the order of the obstacle diameter, are predicted using a second neural network model in series with the first. Together, this serial-modeling approach allows for rapid prediction of flows in domains that can be represented by a disjoint set of structural elements. This type of domain is common, for example, in urban and periurban areas, wherein buildings conform to a common structural motif that affects ground-level velocity fields.
Another relevant length scale is the grid size used to digitize individual domains for read-in by the model. Thus, we investigated how flow patterns can be affected when this input resolution is varied. Although our choice of grid size is somewhat arbitrary, it is dense enough to capture variation in the relevant velocity fields near individual obstacles, but not so dense that producing a large enough cohort of CFD-generated training datasets becomes computationally intractable.
Our approach can also be trained to predict flows with a variable inlet velocity, which, in the case of urban wind flow prediction, permits model parameterization in terms of current meteorological conditions. In the specific case of aerial dispersion of chemicals throughout an urban environment, our predicted flows are considered as the advective field of a drift-diffusion model of molecular dispersion. This advection field plays a central role because concentration fluctuations decorrelate in relationship with the velocity fluctuations of the advection field, and spatial heterogeneity in the flow patterns is determined by the sequence of obstacles in the flow path.