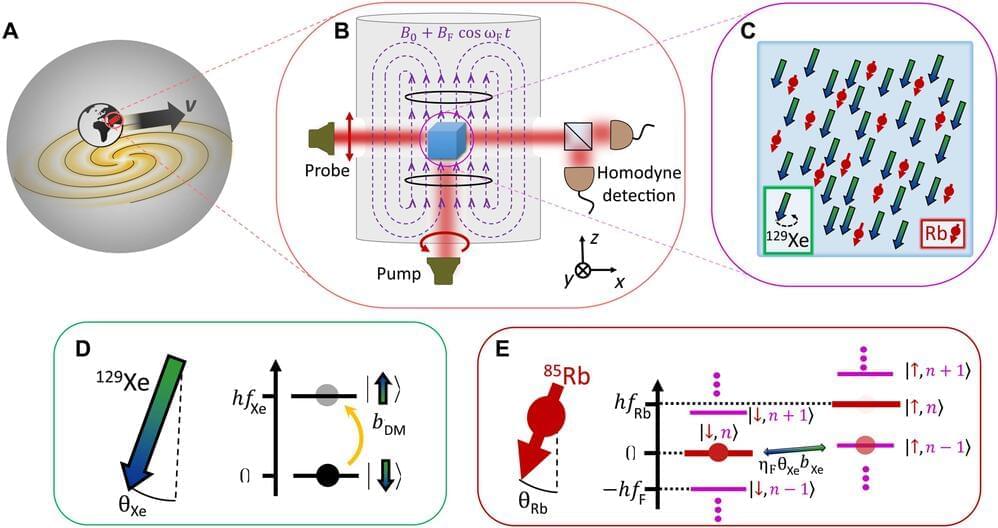
A team of researchers affiliated with several institutions in Israel has used a Floquet quantum detector to constrain axion-like dark matter, hoping to reduce its parameter space. In their paper published in the journal Science Advances, the group describes their approach to constraining the theoretical dark matter particle as a means to learning more about its properties.
Despite several years of effort by physicists around the world, dark matter remains a mystery. Most physicists agree that it exists, but thus far, no one has been able to prove it. One promising theory involving the existence of interacting massive particles has begun to lose its luster, and some teams are looking for something else. In this new effort, the researchers seek axions, or axion-like particles. Such dark matter particles have been theorized to be zero-spin and able to possess any number of combinations of mass and interaction strength. The team sought to constrain the features of axion-like particles to reduce the number of possibilities of their existence and thereby increase the chances of proving their existence.
The researchers used a shielded glass cell filled with rubidium-85 and xenon-129 atoms. They fired two lasers at the cell—one to polarize the rubidium atoms’ electronic spin and the xenon’s nuclear spin, and the other to measure spin changes. The experiment was based on the idea that the oscillating field of the axions would impact on the xenon’s spin when they are close in proximity. The researchers then applied a magnetic field to the cell as a means of blocking the spin of the xenon to within a narrow range of frequencies, allowing them to scan the possible oscillation frequencies that correspond to the range of the axion-like particles. Under this scenario, the Floquet field is theorized to have a frequency roughly equal to the difference between the nuclear magnetic resonance (NMR) and the electron paramagnetic resonance, and their experiment closes that gap.