For the first time ever, an international team of researchers imaged the microscopic state of negative capacitance. This novel result provides researchers with fundamental, atomistic insight into the physics of negative capacitance, which could have far-reaching consequences for energy-efficient electronics.
Category: physics – Page 260
Why it is dangerous to build ever larger big bang machines
Posted in alien life, astronomy, cosmology, energy, engineering, ethics, existential risks, general relativity, governance, gravity, innovation, law, nuclear energy, nuclear weapons, particle physics, philosophy, physics, policy, quantum physics, science, scientific freedom, security, singularity, space travel, supercomputing, theory, time travel | 1 Comment on Why it is dangerous to build ever larger big bang machines
CERN has revealed plans for a gigantic successor of the giant atom smasher LHC, the biggest machine ever built. Particle physicists will never stop to ask for ever larger big bang machines. But where are the limits for the ordinary society concerning costs and existential risks?
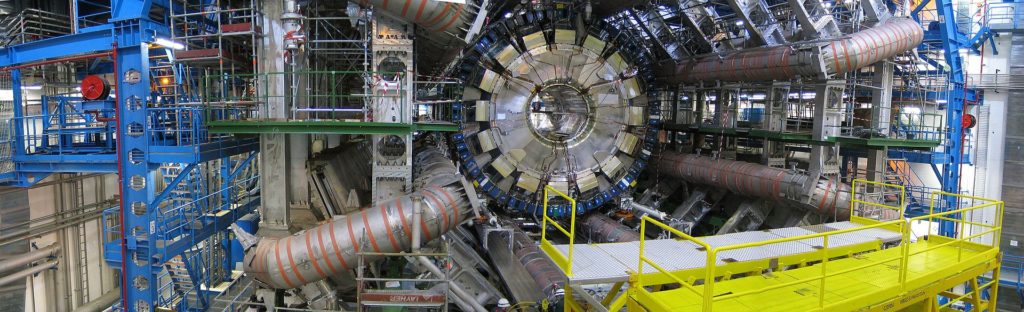
CERN boffins are already conducting a mega experiment at the LHC, a 27km circular particle collider, at the cost of several billion Euros to study conditions of matter as it existed fractions of a second after the big bang and to find the smallest particle possible – but the question is how could they ever know? Now, they pretend to be a little bit upset because they could not find any particles beyond the standard model, which means something they would not expect. To achieve that, particle physicists would like to build an even larger “Future Circular Collider” (FCC) near Geneva, where CERN enjoys extraterritorial status, with a ring of 100km – for about 24 billion Euros.
Experts point out
Wow!
The ability to instantaneously jump from one location in space to another clearly violates the laws of physics. Or does it?
Researchers at the George Washington University have taken a major step toward reaching one of the most sought-after goals in physics: room temperature superconductivity.
Superconductivity is the lack of electrical resistance and is observed in many materials when they are cooled below a critical temperature. Until now, superconducting materials were thought to have to cool to very low temperatures (minus 180 degrees Celsius or minus 292 degrees Fahrenheit), which limited their application. Since electrical resistance makes a system inefficient, eliminating some of this resistance by utilizing room temperature superconductors would allow for more efficient generation and use of electricity, enhanced energy transmission around the world and more powerful computing systems.
“Superconductivity is perhaps one of the last great frontiers of scientific discovery that can transcend to everyday technological applications,” Maddury Somayazulu, an associate research professor at the GW School of Engineering and Applied Science, said. “Room temperature superconductivity has been the proverbial ‘holy grail’ waiting to be found, and achieving it—albeit at 2 million atmospheres—is a paradigm-changing moment in the history of science.”
Physicists have built a ring in which pulses of light whip circles around each other and the normal rules that govern light’s behavior no longer apply.
Under normal circumstances, light displays certain kids of physical symmetry. First, if you were to play a tape of light’s behavior forward and then backward, you would see it behave in the same way moving in both directions in time. This is called time-reversal symmetry. And second, light, which can move through the world as a wave, has what is called polarization: how it oscillates relative to the motion of the wave. That polarization usually stays the same, providing another type of symmetry.
But inside this ring-shaped device, light both loses its time-reversal symmetry and changes its polarization. Inside the ring, light waves turn circles and resonate with one another, producing effects that don’t normally exist in the outside world. [The 10 Most Outrageous Military Experiments].
Dark matter may occasionally interact with minerals in the earth, leaving traces that physicists hope to decipher.
The ¥16.4-billion (US$148-million) observatory — Japan’s Kamioka Gravitational Wave Detector (KAGRA) — will work on the same principle as the two detectors of the Laser Interferometer Gravitational-Wave Observatory (LIGO) in the United States and the Virgo solo machine in Italy. In the past few years, these machines have begun to detect gravitational waves — long-sought ripples in the fabric of space-time created by cataclysmic cosmic events such as the merging of two black holes or the collision of two neutron stars.
LIGO’s Asian cousin will this year deploy ambitious technology to improve sensitivity in the search for these faint, cosmic ripples — but its biggest enemy could be snowmelt.
Just before the new year, a Washington University professor was among a group of scientists who launched a telescope from Antarctica that could observe bright, massive objects in space, like black holes.
The international team of researchers, which included Wash U physics professor Henric Krawczynski, wanted to collect data on black holes and neutron stars, a very dense collapsed core of a giant star.
Studying such celestial phenomena helps astrophysicists test the fundamental laws of physics, Krawczynski said.