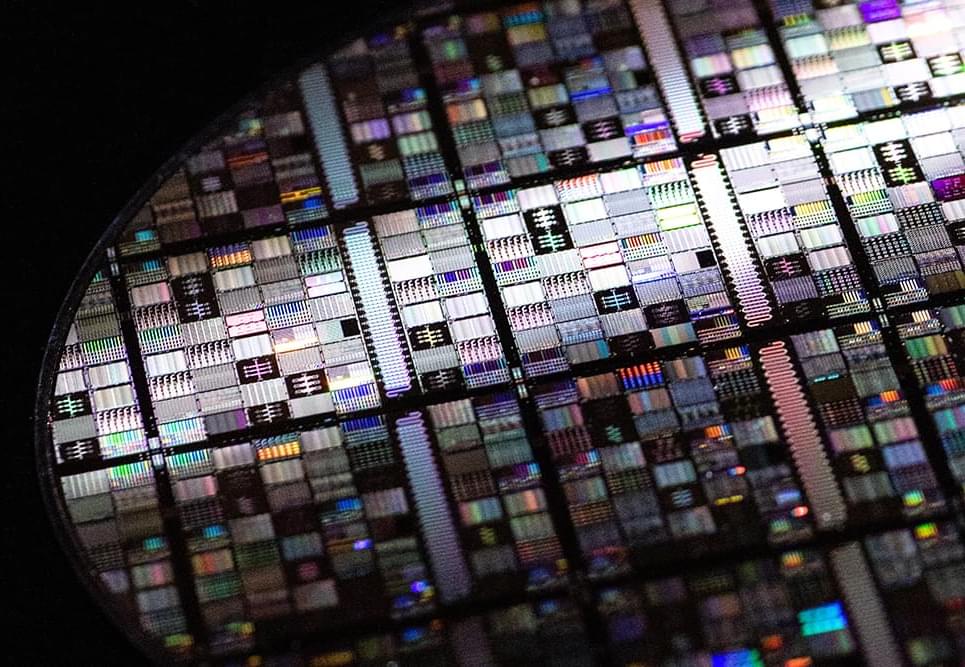
Advancing the state of the art in superconducting qubit hardware requires knowledge across a range of disciplines, including materials, fabrication, circuit design and simulation, packaging, cryogenics, low-noise measurement, hardware-software interfacing, and quantum compilation. As understanding of materials and processes has advanced over time, fabricating the highest-quality qubits increasingly relies on millions of dollars of fabrication equipment and countless hours of process development and sustainment.
“It has become increasingly challenging for individual organizations to maintain this full stack of expertise, particularly as circuits become more complex to design, fabricate, and measure,” Schwartz says. “As a result, superconducting qubit hardware research has remained centralized into a relatively small number of laboratories and large universities capable of developing and sustaining this expertise.”
MIT Lincoln Laboratory is one of these laboratories, with more than 20 years of research and development in superconducting qubits and demonstrations of world-leading qubit performance. The qubits are made on-site at the Microelectronics Laboratory, considered to be one of the U.S. government’s most advanced foundries, and in specialized prototyping facilities. The collective expertise and equipment of this facility have made it possible to stand-up the SQUILL Foundry.